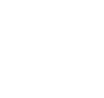
Amyotrophic Lateral Sclerosis (ALS)
Research
Intense research is being conducted in many areas related to ALS, from basic science seeking the roots of the disease to therapy development to find effective treatments.
Since its inception, MDA has dedicated more than $174 million to ALS research.
Research into familial (running in families) forms of the disease also may have relevance for sporadic (nonfamilial) forms, as all ALS cases, regardless of the form, may present and develop along similar lines.
Many other medications and treatments are being tested for potential benefits in ALS.
This section offers an overview and links to more information about ALS research targets and strategies, and research administration in ALS.
Additionally, there are many strategies currently in the pipeline for ALS drug development.
Read about the ALS research MDA has recently funded and also check out recent news about research.
Genetic contributions
Many genes, when mutated, can cause familial ALS. Among them are the SOD1 gene, the TDP43 gene and the FUS gene.1 In 2011, a specific mutation in the C9ORF72 gene was found to be the most common genetic cause of the disease identified so far. Defects in the C9ORF72 gene were also shown to cause another disease called frontotemporal dementia (FTD). Some patients with the gene defect develop ALS, some develop FTD, and some patients develop symptoms of both diseases. (See Causes/Inheritance.) Other genes shown to contribute to rare forms of ALS include ubiquilin 2, profilin 1, valosin-containing protein, alsin, senataxin, angiogenin, and optineurin. If someone with an ALS-causing gene mutation is the first in the family to show the disease, the disorder is classified as sporadic because there is no family history. (This can happen when, for example, a parent carrying the ALS-causing mutation passes away of other causes before ALS develops but not before passing the mutation along to his or her children).2,3
However, research on the genetic factors that contribute to ALS, without necessarily causing it directly, is of great interest. Scientists suspect that a number of gene variants, in combination with other unknown factors, may increase susceptibility to sporadic ALS.
Several large gene association studies have been done comparing the DNA of people with and without ALS in hopes of uncovering genetic differences. These studies have pointed toward potential genetic targets for ALS research.
When scientists studied DNA from 915 people with ALS and 980 without the disease, they found expanded ataxin 2 genes in 43 (4.7%) of those with ALS and only 14 (1.4%) of those without it. They concluded that ataxin 2 expansions are significantly correlated with increased risk for developing ALS. Expanded ataxin 2 protein molecules appear to have toxic interactions with TDP-43, another protein implicated in ALS, and blocking ataxin 2 interactions with TDP-43 is being studied as a new therapeutic avenue.4
There are several approaches in development to treat genetic versions of ALS including familial ALS caused by SOD1 mutations and C9ORF72 expansions. One strategy involves development of a drug called an antisense oligonucleotide (ASO), which is able to block defective SOD1.5 This approach may also be useful for other types of ALS caused by different genes. MDA granted more than $1 million from 2007 to 2012 toward the first clinical trial conducted together with Ionis Pharmaceuticals where the SOD1 ASO was tested. While the first-generation ASO was determined to need adjustments to improve its potency, these early studies were landmark since this was the first trial testing an ASO in neuromuscular disease through intrathecal (injection into the spinal canal) delivery and paved the way for the trials currently in progress. Biogen licensed the SOD1 ASO from Ionis Pharmaceuticals and continued clinical testing of this therapy. In April 2023, the FDA granted accelerated approval to Biogen’s SOD1 ASO therapy (Qalsody) based on secondary endpoints of lowering SOD1 and neurofilament light (NfL) biomarker levels.
Glutamate inhibition
In ALS, there is some evidence that excess amounts of the neurotransmitter glutamate accumulate in the spaces around a nerve cell after it has completed its signaling function, causing problems for nerve cells in the vicinity.
Normally, glutamate, a chemical transmitter of signals between nerve cells, is released by a sending neuron and docks on a receiving neuron. Once docked, it is quickly cleared away by glutamate transporter proteins, which are produced by neighboring cells in the nervous system called astrocytes. In ALS, something may go wrong with this glutamate clearance system. Some studies have suggested that, in ALS, a protein called EAAT2 (a glutamate transporter) may not be as efficient at clearing glutamate away from nerve cells as it should be. Other studies have suggested that a glutamate receptor, a docking site on the surface of motor neurons that receives glutamate, may be excessively permeable in this disease.6
A drug specifically approved for the treatment of ALS by the U.S. Food and Drug Administration (FDA) is riluzole (Rilutek), which is believed to interfere with the action of glutamate. However, other drugs affecting glutamate neurotransmission have not been successful in clinical trials.
Immune system involvement
There is a growing body of evidence that malfunction of the immune system is at least part of the ALS disease process. Research has identified abnormal immune system overactivity in animal models of the disease, and investigators have observed it in blood samples from people with ALS.
It had been thought that motor neurons died on their own in ALS. But, today, there is evidence that immune system cells in the nervous system called microglia are involved in their demise. It’s still unclear whether microglia are helpful or harmful during the disease and microglia may play beneficial/harmful roles at different points during the disease process. 7
Blocking or modifying parts of the immune system is a strategy being pursued in several clinical trials for ALS.
Astrocytes
While some research teams have focused their attention on microglia, the nervous system's immune cells, others have focused more on astrocytes, a type of non-nerve glial cell that normally provides support to motor neurons and other cells in the nervous system. Among their roles is clearing away a potentially toxic compound called glutamate from the area around and between nerve cells. ALS patients have been shown to have dysfunctional clearing of glutamate which is likely because of defects in astrocytes’ activity. These cells acquire pathologic activity, and in experimental models, astrocytes proved to be responsible for motor neuron death.8
Investigators have shown that treating astrocytes alone can delay disease onset and extend survival in mice with a disease resembling ALS caused by mutations in the SOD1 gene.9,10
Currently, there are several laboratories working to understand how astrocytes become dysfunctional in ALS and develop therapies targeting astrocytes in ALS patients.
Misfolded proteins
Cellular proteins normally fold only in certain ways shortly after they're produced. When folding goes wrong, the result may be a highly toxic protein. In 2010, researchers found that misfolded SOD1 protein, unaccompanied by an SOD1 gene mutation, could be found in at least some cases of sporadic ALS.11
Other proteins besides SOD1 may misfold and contribute to the disease as well. For example, the TDP-43 proteins appear to misfold and form clumps (aggregates) in ALS-affected motor neurons even when the genes for these proteins are normal. TDP-43 aggregates have been found in the nervous system tissue for the majority of types of ALS.12 Recent drug development efforts have led to antibody-based therapies targeting misfolded TDP-43, which, if successful, could finally provide a disease-modifying treatment for ALS.
TDP-43 regulates the expression levels of hundreds of RNAs, many of which play important roles in neuronal function. One of these, stathmin-2, appears to be critical for axon regeneration, and altering TDP-43 function prevents stathmin-2 from repairing damaged axons. Because axonal degeneration of motor neurons is a key feature of ALS pathogenesis, rescuing stathmin-2 levels has emerged as a very promising therapeutic target.13
Normally, proteins called chaperones help coax other proteins to fold into the correct shape. Therefore, some scientists are working on increasing levels of these chaperone proteins. Experimental drugs designed to reduce protein aggregation and/or boost chaperone function are being tested in clinical trials for ALS.
Mitochondrial factors
In ALS, the cellular "energy factories" called mitochondria malfunction, although it isn't clear exactly where in the chain of events of the ALS disease process this malfunction occurs.
When mitochondria malfunction, they may fail to produce the needed energy for cells, and they may leak toxic substances called reactive oxygen species, subjecting cells to a kind of poisoning known as oxidative stress.
The U.S. Food and Drug Administration on May 5, 2017, approved edaravone (Radicava) for the treatment of ALS. Radicava is thought to work by relieving the effects of oxidative stress. Targeting this pathway could potentially preserve motor neuron health, which could, in turn, keep muscles functional for a longer period of time. For more, see FDA Approves Radicava to Treat ALSand Questions and Answers About Newly FDA-Approved Radicava to Treat ALS.
Unfortunately, coenzyme Q10, which combats oxidative stress, was not found to be helpful in people with ALS, even at high doses. (Coenzyme Q10 is an antioxidant, which is a substance that helps clean up free radicals.)14
MDA-supported researchers continue to study mitochondrial dysfunction in ALS, with an eye to determining whether it is a cause or a consequence of motor neuron loss and whether restoring mitochondrial function can alter the ALS disease course.
Stem cells
Stem cells can be thought of as cells that are in the very early stages of development, before they become specialized (differentiated) to perform specific roles in tissues. They may be precursors to specific cell types (such as muscle or nerve cells), or they may still retain pluripotency the ability to develop into any cell types.
Stem cell research is an evolving field that may potentially benefit people affected by neuromuscular disease. There are many different types of stem cells used in biological research.
One new type of stem cell is called the induced pluripotent stem cell (iPSC). These stem cells are unique in that they have the regenerative capabilities of embryonic stem cells (they are not created by destroying human embryos). Rather, scientists have discovered methods to create iPSCs in the lab using human skin or blood as a starting source. Induced pluripotent stem cells (iPSCs) have the potential to make most, if not all, tissue types of the body (similar to an embryonic stem cell). Therefore, they are being widely used by scientists to model disease in a dish and are particularly helpful for diseases affecting tissues like the brain and spinal cord (e.g. ALS) mainly since it is difficult to take biopsies of the affected tissues.15
Adult stem cells are a type of stem cell found in all living humans which help to regenerate or repair the body throughout life. Adult stem cells have been found in many tissues including muscle, brain, and bone. These types of stem cells are currently under investigation for their potential to repair, regenerate, and nourish the muscles and nerves damaged in neuromuscular disease.
Stem cells also are in development as cell-transplantation therapies. In ALS, stem cells are being tested in multiple clinical trials for their nourishing abilities to help ailing nerve cells.
Many companies and institutions outside the United States (and a few inside) falsely advertise that they can cure ALS with stem cells. So far, there are no FDA-approved stem-cell-based treatments for ALS, and therefore stem cell therapies should be approached with great caution.
Growth factors
Vascular endothelial growth factor (VEGF) is a signaling protein that stimulates growth of new blood vessels. It has been shown that that patients who have genetic variants in the VEGF gene may be more susceptible to ALS, which has increased enthusiasm for the possible role of growth factors in this disease. Furthermore, studies in a mouse model that does not produce VEFG show that treatment of this animal with VEGF has resulted in significant improvement.16
Apoptosis
Apoptosis, also known as programmed cell death pathway, is an orderly and necessary way of clearing old cells and maintaining balance in the body. In some cases, apoptosis can become dysregulated, and that can lead to disease. Some evidence suggests that apoptosis may be a late pathway for motor neuron degeneration in ALS. There is evidence that inhibition of this programmed cell death pathway in a mouse model of ALS halted neuronal loss and prevented axonal degeneration, symptom onset, and paralysis. Survival was extended in this animal model. These findings suggest that inhibition of apoptosis is a possible therapeutic strategy for ALS.17,18
A wide range of agents targeting different aspects of the pathophysiology of ALS are being explored in both sporadic and familial ALS.
ALS Research Infrastructure
MOVR Data Hub
MDA launched a transformative platform in 2018 called the MOVR Data Hub to capture and aggregate robust clinical data from MDA’s Care Center Network. This clinical data will be married with genetic and patient-reported information to generate a hub of valuable real-world information. Through MOVR (neuroMuscular ObserVational Research), MDA will accelerate the development of new treatments and improve the health outcomes for patients with neuromuscular disease.
MOVR Data Hub ties its origins to the original MDA registry launched in 2013, and has collected clinical data from more than 3,000 patients with ALS, BMD, DMD, and SMA. The pilot phase allowed MDA to gain insights and learn best practices for a multi-disease national neuromuscular disease registry.
Systematic collection and rigorous management of clinical, genetic, and patient-reported data in combination with preparing the community for more clinical trials will power continued research, clinical development, and treatment advancement.
Captured data will be accessible to members of the academic and pharma/biotech communities to enable collaboration and drive better care and more therapies for patients with neuromuscular diseases.
To learn more, click here.
National ALS Registry
In 2010, the U.S. Centers for Disease Control and Prevention opened the National ALS Registry to compile a large database of information about the incidence and prevalence of ALS, how the disease develops, and what types of treatments and interventions are beneficial. Participation in the registry will help researchers understand whether some types of ALS are caused by environmental hazards, geographic exposures, or occupational risks.
People with ALS and/or their caregivers are encouraged to register at cdc.gov/als, where they will be asked to fill out short surveys about their and their families' health, their military background, and their environmental and occupational exposures.
The National ALS Registry has expanded to include a National ALS Biorepository, with the goal to collect and store specimens from ALS patients for use in research studies. Many researchers have limited access to patient specimens. Access to such specimens is crucial because it allows scientists to validate their findings from the lab in actual human samples taken from patients with ALS. The new biorepository will help make more specimens available to researchers that they can use to accelerate therapy development.
Northeast Amyotrophic Lateral Sclerosis (NEALS) Consortium
NEALS is a network of clinics who specialize in ALS clinical trials and research. MDA provides infrastructure support to NEALS to support their biorepository, annual meeting, and genetic testing program. The mission of NEALS is to translate scientific advances into new treatments for people with ALS as rapidly as possible.
To achieve our goal, NEALS functions as an academic research consortium, a contracted research organization, and a resource tool for ALS community.
Clinical Research in ALS and Related Disorders for Therapeutic Development (CReATe) Consortium
CReATe is a Rare Disease Clinical Research Network funded by the National Institute of Health (NIH) with supplemental funding from MDA. The goal of the CReATe Consortium is to advance therapeutic development for sporadic and familial forms of ALS, frontotemporal dementia (FTD), and other related diseases. The CReATe consortium aims to support this goal through study of the relationship between clinical phenotype and underlying genotype, and also through the discovery and development of biomarkers. People with ALS are invited to join CReATe to learn more about CReATe research studies.
References
- Bonne G, Rivier F, Hamroun D. The 2019 version of the gene table of neuromuscular disorders (nuclear genome). Neuromuscul Disord. 2018;28(12):1031-1063. doi:10.1016/j.nmd.2018.09.006
- Nguyen HP, Van Broeckhoven C, van der Zee J. ALS Genes in the Genomic Era and their Implications for FTD. Trends Genet. 2018. doi:10.1016/j.tig.2018.03.001
- Ciura S, Sellier C, Campanari ML, Charlet-Berguerand N, Kabashi E. The most prevalent genetic cause of ALS-FTD, C9orf72 synergizes the toxicity of ATXN2 intermediate polyglutamine repeats through the autophagy pathway. Autophagy. 2016. doi:10.1080/15548627.2016.1189070
- Elden AC, Kim HJ, Hart MP, et al. Ataxin-2 intermediate-length polyglutamine expansions are associated with increased risk for ALS. Nature. 2010. doi:10.1038/nature09320
- McCampbell A, Cole T, Wegener AJ, et al. Antisense oligonucleotides extend survival and reverse decrement in muscle response in ALS models. J Clin Invest. 2018. doi:10.1172/JCI99081
- Kim K, Lee SG, Kegelman TP, et al. Role of Excitatory Amino Acid Transporter-2 (EAAT2) and glutamate in neurodegeneration: Opportunities for developing novel therapeutics. J Cell Physiol. 2011. doi:10.1002/jcp.22609
- Geloso MC, Corvino V, Marchese E, Serrano A, Michetti F, D’Ambrosi N. The dual role of microglia in ALS: Mechanisms and therapeutic approaches. Front Aging Neurosci. 2017. doi:10.3389/fnagi.2017.00242
- Tripathi P, Rodriguez-Muela N, Klim JR, et al. Reactive Astrocytes Promote ALS-like Degeneration and Intracellular Protein Aggregation in Human Motor Neurons by Disrupting Autophagy through TGF-ß1. Stem Cell Reports. 2017. doi:10.1016/j.stemcr.2017.06.008
- Valbuena GN, Tortarolo M, Bendotti C, Cantoni L, Keun HC. Altered metabolic profiles associate with toxicity in SOD1G93A astrocyte-neuron co-cultures. Sci Rep. 2017. doi:10.1038/s41598-017-00072-4
- Silverman JM, Christy D, Shyu CC, et al. CNS-derived extracellular vesicles from superoxide dismutase 1 (SOD1)G93A ALS mice originate from astrocytes and neurons and carry misfolded SOD1. J Biol Chem. 2019. doi:10.1074/jbc.RA118.004825
- Paré B, Lehmann M, Beaudin M, et al. Misfolded SOD1 pathology in sporadic Amyotrophic Lateral Sclerosis. Sci Rep. 2018. doi:10.1038/s41598-018-31773-z
- Joshi AU, Saw NL, Vogel H, Cunnigham AD, Shamloo M, Mochly-Rosen D. Inhibition of Drp1/Fis1 interaction slows progression of amyotrophic lateral sclerosis. EMBO Mol Med. 2018. doi:10.15252/emmm.201708166
- Melamed Z, López-Erauskin J, Baughn MW, et al. Premature polyadenylation-mediated loss of stathmin-2 is a hallmark of TDP-43-dependent neurodegeneration. Nat Neurosci. 2019;22(2):180-190. doi:10.1038/s41593-018-0293-z
- Kaufmann P, Thompson JLP, Levy G, et al. Phase II trial of CoQ10 for ALS finds insufficient evidence to justify phase III. Ann Neurol. 2009. doi:10.1002/ana.21743
- Fujimori K, Ishikawa M, Otomo A, et al. Modeling sporadic ALS in iPSC-derived motor neurons identifies a potential therapeutic agent. Nat Med. 2018. doi:10.1038/s41591-018-0140-5
- Keifer OP, O’Connor DM, Boulis NM. Gene and protein therapies utilizing VEGF for ALS. Pharmacol Ther. 2014. doi:10.1016/j.pharmthera.2013.10.009
- Rabizadeh S, Gralla EB, Borchelt DR, et al. Mutations associated with amyotrophic lateral sclerosis convert superoxide dismutase from an antiapoptotic gene to a proapoptotic gene: studies in yeast and neural cells. Proc Natl Acad Sci U S A. 1995.
- Guégan C, Przedborski S. Programmed cell death in amyotrophic lateral sclerosis. J Clin Invest. 2003. doi:10.1172/jci200317610